Michael A Freed
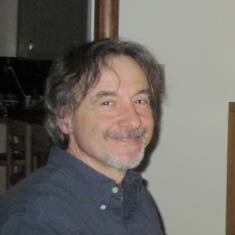
Research Associate Professor
Department of Neuroscience
University of Pennsylvania
Perelman School of Medicine
Philadelphia, PA 19104
Foreword
Understanding the retina is like understanding an electronic circuit except for one important difference. Someone, somewhere, designed the electronic circuit. The retina is different because no one (arguably) designed it, at least not anyone who can explain it to us. The retina is like an electronic circuit whose schematics have been lost, whose components have unknown specifications, and whose design is a matter of conjecture. Therefore my lab studies retinal components to determine their specifications, and integrates this knowledge into systems-level hypotheses about the overall design of the retina.
Noise generated by chemical synapses
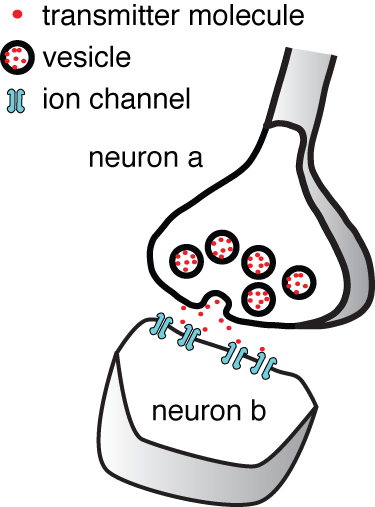
Synaptic noise Vesicles in cell a fuse with its membrane and release transmitter onto cell b. We asked how much of the noise in the postsynaptic cell b originates from random fusion of synaptic vesicles.
One can read in any Neuroscience textbook about how a synapse transmit information from one cell to another. A vesicle fuses with the membrane of a neuron a, and releases its fill of neurotransmitter onto the membrane of neuron b. Yet it is not commonly appreciated how noisy and thus unreliable synaptic transmission is. Synaptic transmission is noisy because the fusion of a vesicle depends on small numbers of molecules: only 100's of calcium ions, 10's of calcium channels, and 10's of SNARE complexes. Once transmitter is released from a vesicle, there are only 10's of postsynaptic receptors to bind it. Small numbers of molecules mean that random Brownian motion and random molecular conformational changes become important. Surprisingly, the amount of noise a synapse generates had not been studied.
Our approach is to record ionic currents generated in the postsynaptic cell and then subject them to noise analysis. Noise analysis makes use of a mathematical relationship between mean current (µ), the variance of this current (σ2), and the event rate (n): (µ2 = σ n T), where T depends on the shape of a randomly occuring event. For the ionic currents, the random event is the postsynaptic current caused by the fusion of a single vesicle. Thus we can estimate the noise variance generated by vesicle fusion given that we know the values for the other parameters.
So far, we have focused on ionic currents recorded from retinal ganglion cells and analyzed noise generated by bipolar cell synapses. We have found that much of the noise in these currents— about 40% — comes from vesicle fusion, very little from the ganglion cell itself, and most of it — about 60% — is generated by the presynaptic cell and circuit component that feed information to the presynaptic cell. Thus we have come to the conclusion that the bipolar cell synapse adds a signficant amount of noise to the information it transmits. Our approach can be used for other synapses in the retina, and this is what we plan to do.
Reverse engineering retinal design
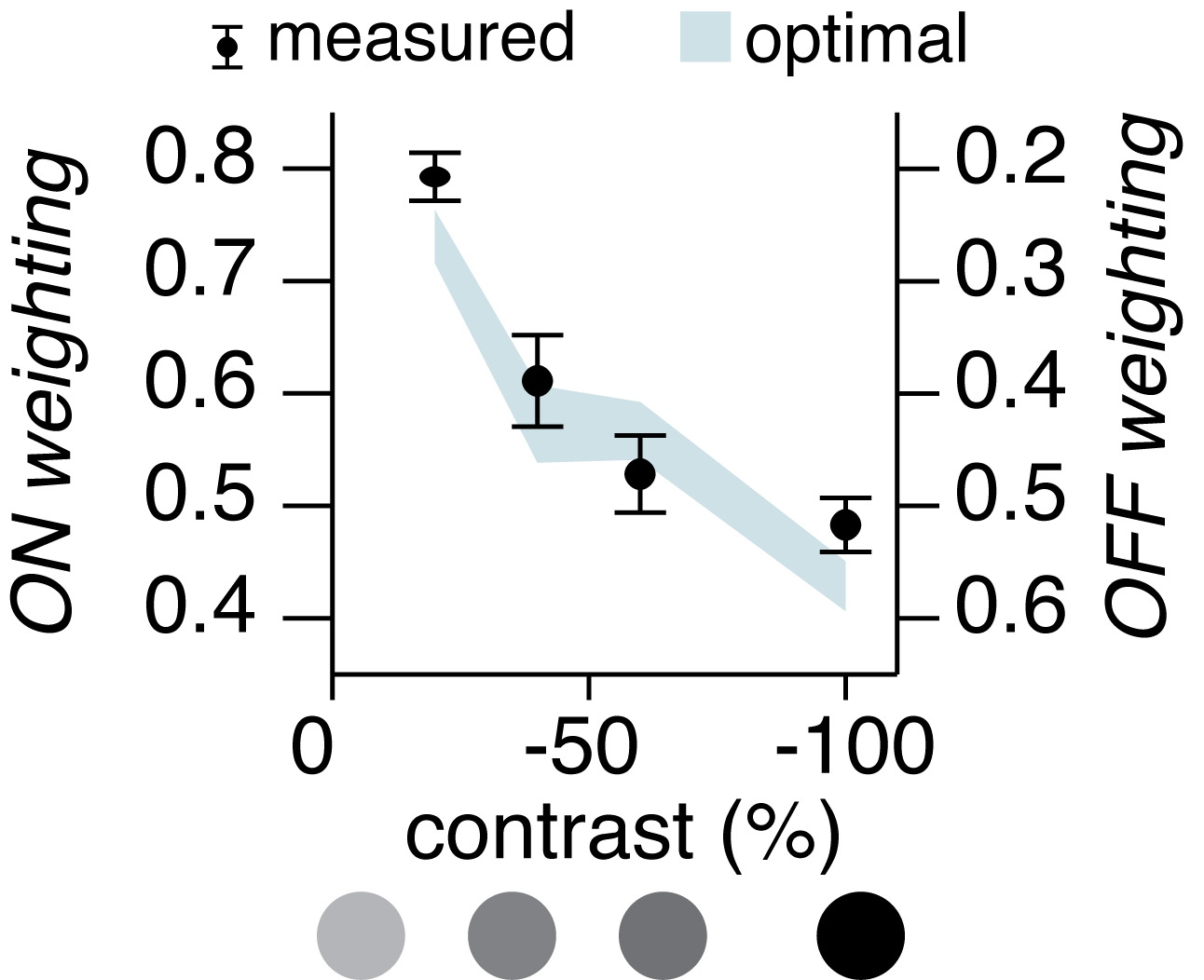
The weighting of ON and OFF inputs to an OFF alpha retinal ganglion cell. Weighting is expressed on a scale of 0 to 1. Here we compare actual weighting to what is optimal according to Bayesian reasoning. Note that actual and optimal weightings are a good match.
Don't believe everything you read — or so the aphorism goes. When confronted with many sources of information, the best advice is to give greater weight to the reliable source. The brain needs to do this everyday because objects are apprehended using more than one sense: taste, smell, touch, sight, and sound. Neurons also receive information through their synapses from multiple sources, sometimes from multiple senses. And so we asked whether neurons weigh information according to reliability.
We asked this question of the retina because after the photoreceptors convert light into electrical signals, the perceptions of light and dark are divided into separate circuits, labeled ON and OFF. These circuits run separately for the most part, but here and there they converge onto single neurons. We chose one of these neurons to study, the OFF alpha cell. This cell receives ON and OFF information through separate synapses.
We found that indeed the weighting of ON and OFF information is according to reliability. As one might guess, the ON stream does not signal a dark black spot very reliably, but signals a moderately grey spot somewhat more reliably. Correspondingly, as the spot grew darker, the ON synaptic input grew weaker and the OFF synaptic input grew stronger. Therefore, the cell weighted synaptic inputs by their reliability, and this weighting was dynamically readjusted as the visual stimulus changes.
Apparently this retinal circuit follows the algorithm: weight information by its reliability. We are facinated to gain this glipse of retinal design and plan to pursue it further in the hopes of understanding how different types of synapses fit into the overall design.
How do specific components fit into retinal design?
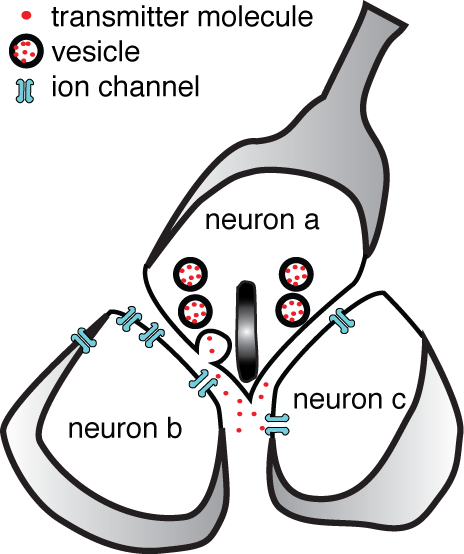
At a dyadic synapse a single vesicle from cell a can release transmitter that binds to receptors on cells b and c.
Often when we try to understand the retinal circuit, we are confronted by a circuit component whose specifications are extraordinary, whose placement in the circuit cannot be serendipitious, but whose purpose is unclear. The dyadic synapse is one such component. At a dyadic synapse, one neuron releases transmitter onto two postsynaptic neurons. A dyadic synapse always contains a structure called a ribbon or button, although not all ribbon/button synapses are dyadic. It is apparent that a dyadic synapse would allow a single synaptic vesicle to create an ionic current in two postsynaptic neurons, but if and why this is necessary to the design of the retina is not clear.
To investigate the dyadic synapse, we use a mouse whose DNA incorporates a jellyfish gene that codes for a green fluorescent protein (eGFP). The protein is expressed by one type of retina ganglion cell, allowing us to microscopically target pairs of ganglion cells where both members of the pair are of the same type. We can then voltage clamp the two cells and record their ionic currents. The two cells are postsynaptic to the same dyadic synapse, and thus we plan to determine if the dyadic synapses convey any special properties to their responses.